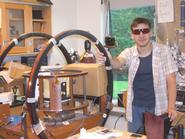
In researching and experimenting with magnetic properties, scientists use beams of neutrons with all the same spin. They ensure that the neutrons are all polarized the same way with the help of an apparatus called a helium-3 polarizer. Jake Zappala ’12 is engineering a helium-3 polarizer test system for researching the diagnostic tools used in the polarization process.
Although magnetic fields and the polarized neutron beams used to test them seem disconnected from our everyday lives, it’s impossible to avoid magnetic fields. One of the main ways that engineers use polarized neutron beams is to test the magnetic properties of hard drives, enabling them to make smaller, faster hard drives that work better in your computer.
Neutrons, like all particles, carry an intrinsic property called spin, which determines their magnetic polarization. The neutron beams researchers use for their experimentation, however, provide unpolarized beams with both up and down spin neutrons. To achieve polarization, (i.e. to get all the neutron spins pointing in the same direction), the beam goes through a glass cell filled with polarized helium-3 gas, which absorbs neutrons of only one spin, allowing only neutrons of the other spin to go through. This finally creates a polarized neutron beam.
But the creation of polarized helium-3 in the cell of the polarizer is quite complicated. To polarize the helium, scientists must first polarize a small amount of rubidium by heating the cell to 170 degrees Celsius, turning some of the rubidium into its gas form, and polarizing it by use of a laser. The rubidium then transfers its polarization to the helium-3, and goes back to its original state. At this point the laser polarizes it again, and the process continues on. The apparatus is also fitted with two sets of coils, one that creates a primary magnetic field for polarization purposes and another (called RF coils) that allow the researchers to run diagnostics and fine-tune the magnetic field. However, despite theoretically being able to go further, scientists have only been able to achieve a certain level of partial polarization of the helium-3 for reasons that are still unknown.
In searching for what the problem is, Zappala is attempting the daunting task of engineering a helium-3 polarizer that safely enables all of these processes. Guided by Associate Professor of Physics Brian Collett, Zappala is starting by installing all of the components necessary to run an extremely uniform magnetic field (with an error of only one part in ten thousand). He is then building a heater that allows researchers to set the temperature required for optical pumping. Simultaneously, he is designing the both the RF coils and the oven, which contains the cell and offers the ability to monitor the cell with various diagnostic tools. Afterward, if he has time, Zappala will set up the system of lasers and optics/mirrors that are used to polarize the rubidium.
But, most importantly, Zappala is learning that in his engineering and design size really does matter—both practically and scientifically. He has to make sure all the components are the right size to fit in their place in the apparatus, but also that they are they are large enough (or small enough) to provide the right magnetic fields without suffering interference or overheating the system.
Hamilton already has a Helium-3 polarizer, but Zappala’s will hopefully have a higher rate of polarization as well as offering an opportunity to test cells of different styles and qualities, which is the ultimate goal of having another polarizer. “By testing new cells, we’re going to end up exploring what could be wrong with them,” Zappala said. “Building new cells could solve the problem of not being able to reach a high level of helium polarization.”
Zappala is a physics and creative writing double major.
Zappala is a graduate of Regis High School on the Upper East Side in Manhattan
Although magnetic fields and the polarized neutron beams used to test them seem disconnected from our everyday lives, it’s impossible to avoid magnetic fields. One of the main ways that engineers use polarized neutron beams is to test the magnetic properties of hard drives, enabling them to make smaller, faster hard drives that work better in your computer.
Neutrons, like all particles, carry an intrinsic property called spin, which determines their magnetic polarization. The neutron beams researchers use for their experimentation, however, provide unpolarized beams with both up and down spin neutrons. To achieve polarization, (i.e. to get all the neutron spins pointing in the same direction), the beam goes through a glass cell filled with polarized helium-3 gas, which absorbs neutrons of only one spin, allowing only neutrons of the other spin to go through. This finally creates a polarized neutron beam.
But the creation of polarized helium-3 in the cell of the polarizer is quite complicated. To polarize the helium, scientists must first polarize a small amount of rubidium by heating the cell to 170 degrees Celsius, turning some of the rubidium into its gas form, and polarizing it by use of a laser. The rubidium then transfers its polarization to the helium-3, and goes back to its original state. At this point the laser polarizes it again, and the process continues on. The apparatus is also fitted with two sets of coils, one that creates a primary magnetic field for polarization purposes and another (called RF coils) that allow the researchers to run diagnostics and fine-tune the magnetic field. However, despite theoretically being able to go further, scientists have only been able to achieve a certain level of partial polarization of the helium-3 for reasons that are still unknown.
In searching for what the problem is, Zappala is attempting the daunting task of engineering a helium-3 polarizer that safely enables all of these processes. Guided by Associate Professor of Physics Brian Collett, Zappala is starting by installing all of the components necessary to run an extremely uniform magnetic field (with an error of only one part in ten thousand). He is then building a heater that allows researchers to set the temperature required for optical pumping. Simultaneously, he is designing the both the RF coils and the oven, which contains the cell and offers the ability to monitor the cell with various diagnostic tools. Afterward, if he has time, Zappala will set up the system of lasers and optics/mirrors that are used to polarize the rubidium.
But, most importantly, Zappala is learning that in his engineering and design size really does matter—both practically and scientifically. He has to make sure all the components are the right size to fit in their place in the apparatus, but also that they are they are large enough (or small enough) to provide the right magnetic fields without suffering interference or overheating the system.
Hamilton already has a Helium-3 polarizer, but Zappala’s will hopefully have a higher rate of polarization as well as offering an opportunity to test cells of different styles and qualities, which is the ultimate goal of having another polarizer. “By testing new cells, we’re going to end up exploring what could be wrong with them,” Zappala said. “Building new cells could solve the problem of not being able to reach a high level of helium polarization.”
Zappala is a physics and creative writing double major.
Zappala is a graduate of Regis High School on the Upper East Side in Manhattan
Posted August 4, 2010